Voices of U of U Health
Bringing Pediatric Oncology into the Future
Cancer care is complex. A drug dose that works perfectly for one person may be completely ineffective for another. One patient may develop long-term organ damage due to unanticipated toxicity, while another may not. For children with cancer who experience complex drug regimens, drug safety and efficacy are challenging.
And if we can’t predict what a drug might do to a given patient, how can we personalize cancer care?
A new digital pharmacology platform is addressing the root of this issue to bring pediatric cancer care into the future. We are on the path to filling knowledge gaps and advancing therapeutic drug use for children with cancer.
Complications of Cancer Care
Ensuring chemotherapy use is as safe and effective as possible is critical to pediatric oncology. This is also true for non-chemotherapy drugs, called supportive care medications.
Supportive care medications make up over 90% of the drugs that children with cancer receive. They treat or prevent conditions like pain, infection, and nausea. The keys to ensuring safe and effective drug use include:
- What the body does to a drug (pharmacokinetics or PK)
- The drug’s effects on the body (pharmacodynamics or PD)
The catch is that other drugs, age, organ function, and disease state can change a drug’s PK and, consequently, its effects. On top of this, very little PK and PD data specific to children with cancer are available.
Even minor genetic differences in genes responsible for drug metabolism can produce profound alterations in drug PK.
In this way, two children with similar characteristics (e.g. age and weight, which are what most dosing is based on) can react differently to the same drug and dose. And both children may react differently than an adult would.

How Patients and Treatments Differ
In cancer treatment, critical issues of PK and PD are often not well understood. Will coinciding supportive care medications influence the PK or PD of chemotherapeutics? Are children with cancer more susceptible to certain drug toxicities? These are some of the unknowns we face.
There are huge gaps in the pediatric cancer literature about how drugs might interact with one another, how drug metabolism changes in different disease states, and what drug target concentrations are best suited to achieve optimal therapeutic outcomes.
We need to better understand these questions, even for drugs that have been in use for decades.
Acetaminophen is one example of a commonly used drug that may put children with cancer at increased risk for toxicity compared to their cancer-free peers.
Normally, the liver safely metabolizes acetaminophen by several pathways. But in children with cancer, these “safe” pathways may become depleted, overwhelmed, or both. This can cause acetaminophen metabolism to be shunted into a pathway that creates a toxic substance, causing liver damage.
These metabolic pathways can differ for several reasons: poor nutrition, high medication burden, other liver toxic drugs (e.g., chemotherapeutics), and increased enzyme expression in the “toxic pathway.” These factors are more common among children with cancer.
Pediatric cancer survivors often suffer long-term liver damage. Using our pharmacology platform, we can evaluate patient- and clinical-level risk factors, acetaminophen and metabolite PK, and biomarkers for liver toxicity.
Ultimately, a better understanding of acetaminophen PK and risk factors for toxicity helps us make informed adjustments in dosing. Thereby, minimizing risk of harm and improving pain management.
Addressing Unknowns
To reiterate, cancer care (especially pediatric care) is incredibly complicated. Ideally, clinicians could use population-specific PK and PD information to tailor dosing to individual needs.
But this information is often lacking for children with cancer. By generating population-specific PK and PD data, we can aid prescribers in making challenging clinical decisions.

The PK Platform
I set out to address the gaps in our knowledge about drugs used to treat children with cancer—and thereby to improve how we apply them in pediatric oncology.
My lab created a sophisticated platform that can generate PK and PD data for niche populations.
Here is how it works.
Resourceful Sampling
The first issue we faced was getting representative samples from very specific populations.
Our solution was to use an approach called opportunistic or scavenge sampling. In our case, we collect scavenge blood samples that were collected as part of standard of care. That is, a physician orders a blood draw for clinical testing, after which excess blood is discarded.
But because these samples are already in our hospital system, our lab can “scavenge” excess blood targeted for discard and use this to conduct our studies—without any added burden to the patient of additional blood draws.
In pharmacology, this process is known as “sparse sampling.” Sparse sampling is ideally suited to current PK modeling approaches. PK and PD modeling using sparse sampling can be used to determine patient-related factors that define drug PK, safety, and efficacy relationships. PK models can also be used to predict accurate descriptions for the PK of a drug in an individual patient.
Finding the “right” scavenge blood samples for our sparse sampling strategy was a bit like finding a needle in a haystack. We needed blood collected at specific times, related to when a drug was administered. These times vary by PK characteristics of the drug and the route by which it was administered.
My team developed software programs that integrate with hospital electronic medical records and our biobank database to identify priority target specimen requests. At present, we can select samples based on:
- Patient demographics
- Diagnoses
- Prescribing information
- Blood collection information
- Coinciding medications
- Lab values for a given patient
Our process allows us to efficiently target samples for collection. We can also assess progress on our collection targets to taper collection or identify where we are lacking.
The platform facilitates a variety of PK drug studies, spanning commonly to rarely prescribed drugs. So far, every study has yielded important clinical insights.

Targeted Analysis
Beyond analyzing blood specimens for drug and metabolite concentrations, we can analyze other things found in patients’ blood, like DNA and biomarkers of drug toxicity.
There are two facets to how we analyze samples.
The first facet is all about pharmacokinetics. By looking at drug concentrations, we can see how patients metabolize drugs and whether we are achieving therapeutic concentration ranges associated with efficacy in general.
Our first goal is to understand whether clinical targets are met. By evaluating if there are patients who fall outside therapeutic ranges, we can tease apart the underlying reasons why. Right now, we are filling gaps in our understanding of how drugs behave to optimize how we use them.
The second facet takes sampling further. Patient specimens provide us with real world blood concentrations. We can translate these drug concentrations to “bench-based” in vitro systems. This provides valuable biological insight.
For example, we are evaluating novel epigenetic mechanisms between supportive care medications and cancer cells and how these may impact chemotherapeutic efficacy.
Unraveling these complex interactions is crucial to personalizing cancer care.
Playing the Long Game
This platform is still very new. We are building a data pool to fill in major knowledge gaps about current therapeutic drug use. We are also preparing for the inevitable evolution of drug therapy as new medications are introduced.
As the platform becomes more sophisticated, we will be able to answer more specific questions. For instance, with growing amounts of data, we can more confidently simulate how specific drugs might behave in each patient.
Personalized dosing is critical in pediatric oncology. And this platform begins to give us the power to do it.
Our long-term goal is completely individualized care. That means accounting for a patient’s genetics to tailor treatment and increase efficacy. It means individualized dosing for every supporting drug a patient takes.
Going Global
This is not just about Huntsman Cancer Institute at the University of Utah, U of U Health, Utah, or even the U.S. Our aim is to improve clinical care globally.
As we grow, our goal is to make this platform available to other cancer researchers and clinicians. I’m excited to see where the future takes us as we increase our scope. Our mission is to have the broadest impact possible and improve cancer care for children everywhere.
And that’s a tall order. But right now, we are demonstrating that it can be done.
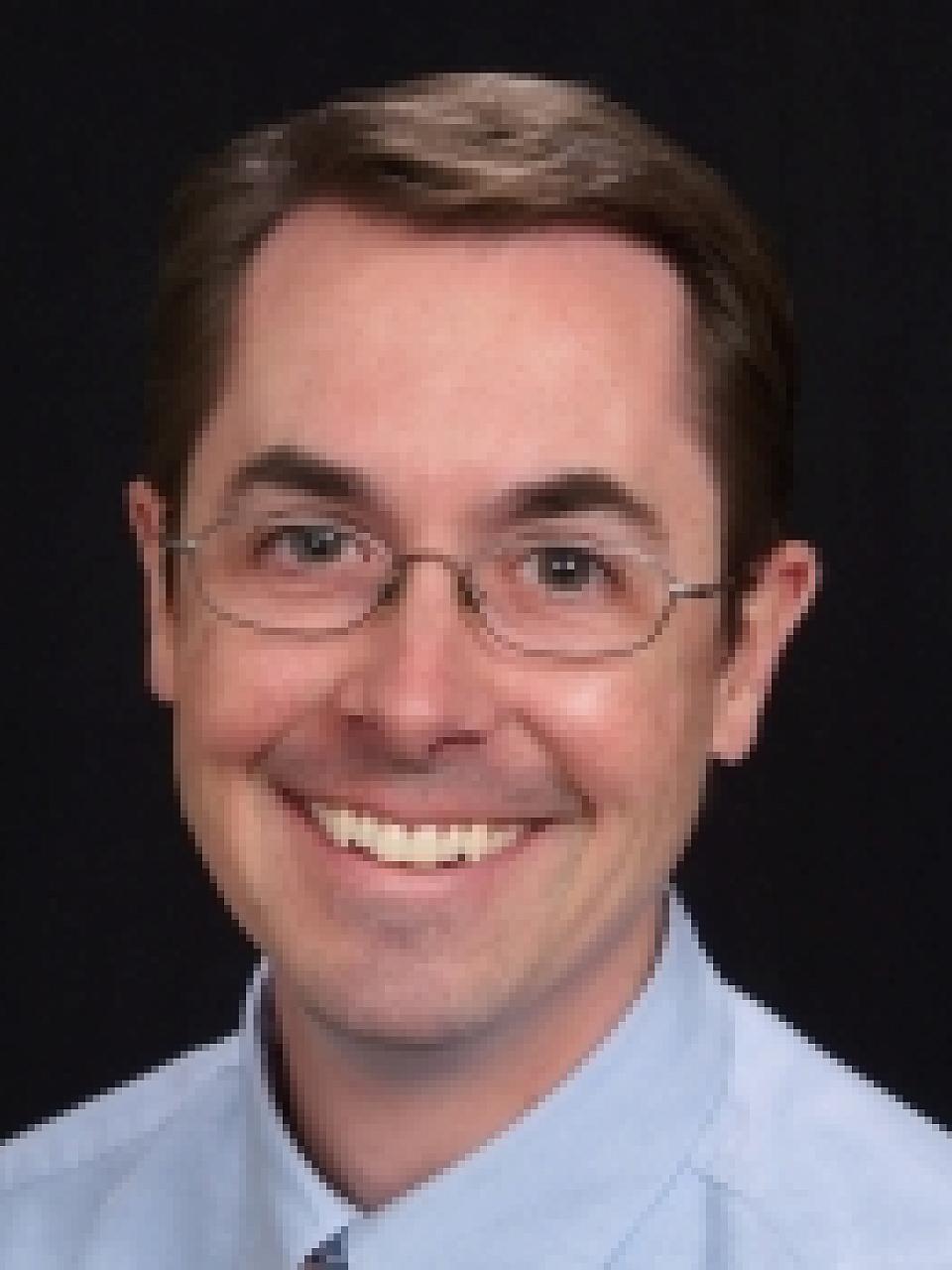